THE HEART
www lamission edu/lifesciences/lecturenote/AliPhysio1/Heart pdf
larger blood volume in exercising conditions) ? Note: Coronary arteries (from the first branching of aorta) supply oxygenated blood to the cardiac muscle
Anatomy of the Human Heart
eknygos lsmuni lt/springer/675/51-79 pdf
The internal anatomy of the heart reveals four chambers 1999 Biological Sciences Textbooks, Inc This material is used by permission of John Wiley
Biology Study Guide 1 - Blood Flow in the Heart pdf
www sac edu/StudentServices/EOPS/Documents/Biology 20Study 20Guide 201 20- 20Blood 20Flow 20in 20the 20Heart pdf
BLOOD FLOW IN THE HEART 1 Blood enters right atrium from superior and inferior vena cavae 2 Blood in right atrium flows through right AV valve
Circulatory System
www uc edu/content/dam/uc/ce/docs/OLLI/Page 20Content/OLLI 20Circulatory_System pdf
circulatory system delivers oxygen and nutrients to your heart and of the high pressure of blood coming from the heart Bio-Concave as they have
Biology Cardiovascular System: Building a Functioning Heart
www stemillinois com/images/heartscard pdf
Biology Cardiovascular System: Building a Functioning Heart Related Standards: Materials: ? Food colouring ? Tape ? Modeling clay or play dough
body fluids and circulation chapter 18 - NCERT
ncert nic in/textbook/ pdf /kebo118 pdf
BIOLOGY You have learnt that all living cells have to be provided with nutrients, circulatory system in which the blood pumped by the heart is always
10-Biology pdf - Class Notes
opjsrgh in/Content/Worksheet/PRACTICE-WS/2021-2022/day53/10-Biology pdf
The human circulatory system consists of a network of arteries, veins, and capillaries, with the heart pumping blood through it The primary role of the
GCSE Review 1 – The Heart & Cardiovascular System
www reigate ac uk/wp-content/uploads/2020/05/1 -The-Heart-Cardiovascular-System pdf
Why do the labels for the right and left sides of the heart appear on the wrong sides? of the A-level Biology course In order to feel even more
LIFE PROCESSES Class 10 Biology TRANSPORTATION PART 1
www aees gov in/htmldocs/downloads/e-content_06_04_20/X_Life_processes_Transportation__Module_1_of_4 pdf
system in place to ensure that this network can be repaired if damaged PARTS OF THE CIRCULATORY SYSTEM / THE CARDIOVASCULAR SYSTEM 1) THE HEART 2) BLOOD 3)
Systems biology approaches to heart development and congenital
academic oup com/cardiovascres/article- pdf /91/2/269/827841/cvr126 pdf
28 avr 2011 systems biology, started to complement the single-gene focus in the Systems biology † Heart development † Congenital heart disease
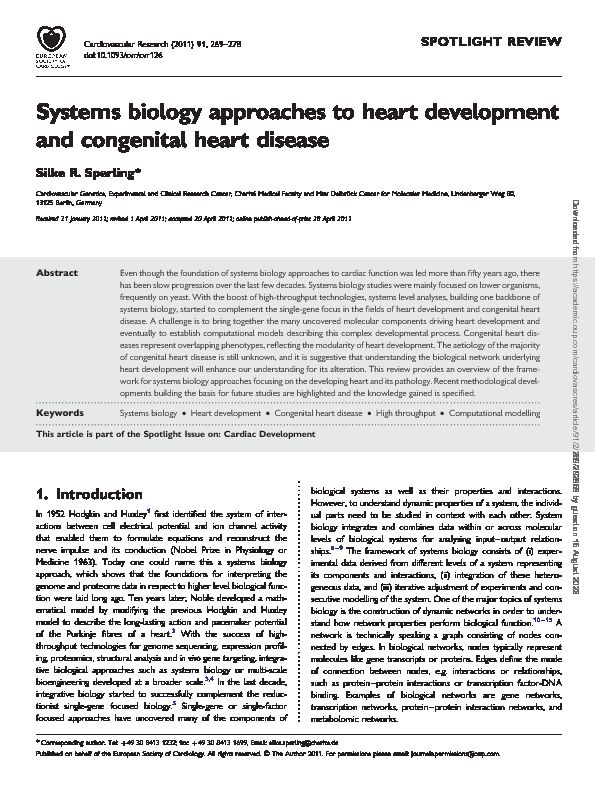
32039_7cvr126.pdf
SPOTLIGHT REVIEW
Systems biology approaches to heart development
and congenital heart disease
Silke R. Sperling
*
Cardiovascular Genetics, Experimental and Clinical Research Center, Charite´Medical Faculty and Max Delbru¨ck Center for Molecular Medicine, Lindenberger Weg 80,
13125 Berlin, Germany
Received 21 January 2011; revised 1 April 2011; accepted 20 April 2011; online publish-ahead-of-print 28 April 2011
AbstractEven though the foundation of systems biology approaches to cardiac function was led more than fifty years ago, there
hasbeenslowprogression overthe last fewdecades. Systems biologystudiesweremainly focusedon lowerorganisms,
frequently on yeast. With the boost of high-throughput technologies, systems level analyses, building one backbone of
systems biology, started to complement the single-gene focus in the fields of heart development and congenital heart
disease. A challenge is to bring together the many uncovered molecular components driving heart development and
eventually to establish computational models describing this complex developmental process. Congenital heart dis-
eases representoverlapping phenotypes, reflecting the modularity of heart development. The aetiologyof the majority
of congenital heart disease is still unknown, and it is suggestive that understanding the biological network underlying
heart development will enhance our understanding for its alteration. This review provides an overview of the frame-
workforsystemsbiologyapproachesfocusingonthedevelopingheartand itspathology.Recent methodologicaldevel-
opments building the basis for future studies are highlighted and the knowledge gained is specified.-----------------------------------------------------------------------------------------------------------------------------------------------------------
KeywordsSystems biologyHeart developmentCongenital heart diseaseHigh throughputComputational modelling
-----------------------------------------------------------------------------------------------------------------------------------------------------------
This article is part of the Spotlight Issue on: Cardiac Development
1. Introduction
In 1952 Hodgkin and Huxley
1 first identified the system of inter- actions between cell electrical potential and ion channel activity that enabled them to formulate equations and reconstruct the nerve impulse and its conduction (Nobel Prize in Physiology or Medicine 1963). Today one could name this a systems biology approach, which shows that the foundations for interpreting the genome and proteome data in respect to higher level biological func- tion were laid long ago. Ten years later, Noble developed a math- ematical model by modifying the previous Hodgkin and Huxley model to describe the long-lasting action and pacemaker potential of the Purkinje fibres of a heart.2
With the success of high-
throughput technologies for genome sequencing, expression profil- ing, proteomics, structural analysis andin vivogene targeting, integra- tive biological approaches such as systems biology or multi-scale bioengineering developed at a broader scale. 3,4
In the last decade,
integrative biology started to successfully complement the reduc- tionist single-gene focused biology. 5
Single-gene or single-factor
focused approaches have uncovered many of the components ofbiological systems as well as their properties and interactions.
However, to understand dynamic properties of a system, the individ- ual parts need to be studied in context with each other. System biology integrates and combines data within or across molecular levels of biological systems for analysing input-output relation- ships. 6-9 The framework of systems biology consists of (i) exper- imental data derived from different levels of a system representing its components and interactions, (ii) integration of these hetero- geneous data, and (iii) iterative adjustment of experiments and con- secutive modelling of the system. One of the major topics of systems biology is the construction of dynamic networks in order to under- stand how network properties perform biological function.10-15 A network is technically speaking a graph consisting of nodes con- nected by edges. In biological networks, nodes typically represent molecules like gene transcripts or proteins. Edges define the mode of connection between nodes, e.g. interactions or relationships, such as protein-protein interactions or transcription factor-DNA binding. Examples of biological networks are gene networks, transcription networks, protein-protein interaction networks, and metabolomic networks. *Corresponding author. Tel:+49 30 8413 1232; fax:+49 30 8413 1699, Email: silke.sperling@charite.de Published on behalf of the European Society of Cardiology. All rights reserved. &The Author 2011. For permissions please email: journals.permissions@oup.com.
Cardiovascular Research (2011)91, 269-278
doi:10.1093/cvr/cvr126Downloaded from https://academic.oup.com/cardiovascres/article/91/2/269/292858 by guest on 15 August 2023
This review attempts to give an overview of the developing field of cardiac systems biology with a focus on heart development. Although .3000 publications of systems biology studies exist in PubMed cur- rently, only a limited number of these refer to cardiogenesis, which is conceptional and experimental challenging due to the complexity of this developmental process involving multiple cell lineages and diverse layers of regulation. The mammalian heart is composed of diverse cell types and structures such as cardiac and smooth muscle cells contributing to the contractile apparatus and vascular system, the conduction system as well as endothelial, valvular, and interstitial mesenchymal fibroblast cells. During evolution the heart developed from a two-chambered organ in fish 16 to a four-chambered one in mammals, 17,18 where its anatomy enables the separation of low- and high-oxygen blood flow. Along with this macro-structural devel- opment a full panel of changes in its physiology occurred. It is highly suggestive that the interaction between environmental factors (e.g. physiological conditions such as oxygen content or pressure load) and the molecular makeup (e.g. transcript levels, protein modifi- cations, protein-protein interactions) of the cardiomyocytes has played a crucial role in this evolutionary developmental process, which is in part mimicked during the embryonic development of the vertebrate heart. The study of heart development is naturally confronted with very limited material for experimental research. At birth 1 mm 3 of heart muscle in rat consists of?3.3×10 5 cardiomyocytes and a mouse heart at E11.5 consists of?1×10 6 cells, including all different cell types and cardiac structures. Therefore, the development of methods suitable to gather sufficient tissue- and cell-selective material (e.g. ES or iPS cells, GFP marked cells) and the establishment of tech- nologies enabling the study of limited biological material up to single cells is essential to systems biology approaches of heart develop- ment. 19-24 A crucial issue remains in the application of high- throughput phenotyping technologies starting from static phenotyping at a cellular level (e.g. histology) up to the study of dynamic functional parameters (e.g. pressure load, shear stress, oxygen supply, and heart beat) underin vivoconditions. 25-29
Haemodynamic and metabolic
factors impact on the molecular portrait of cardiomyocytes. 30
In addition, environmental and hormonal factors like vitamins (folic acid), high carbon levels (diabetes), or high-fat diet are well known to influence the developmental process and enhance the risk of car- diovascular disease. 31-33
To fully understand heart development and eventually its aberra- tion and dysfunction, integrated multi-scale multi-functional models will need to be developed, which represents an ambitious aim and is envisaged by the Cardiac Physiome project. 34
If we can gain insights
even into a subset of functional relationships and circuits between molecular data (-omics data), physiological function and structural development, significant insights into clinically important aspects of human heart disease will follow. Systems biology approaches can be applied at different scales to investigate a key aspect of cardiomyocyte physiology like the transcriptome or a particular biochemical pathway, or to model cardiomyocte function as a whole. Yet it is obvious that the current framework for considering heart development, based on the combinatorial action of tissue-restricted transcription factors, is incomplete and approaching heart development and disease in a systems biology manner has the promise to enable the identification of the missing pieces of the network and moreover to predict pheno- typic outcome.
2. The framework of systems
biology approaches to heart development Systems biology is an integrated approach and, therefore, requires a distinct set of tools, namely an applicable model system enabling per- turbations, suitable phenotype assessment, biotechniques measuring molecular states, and interactions; and computational methods and resources providing the analysis platform for probabilistic and math- ematical modelling (Figure1).
2.1 Applicable biological systems
2.1.1 Animal models
Zebrafish (Danio rerio) is a valuable model for the study of heart for- mation as it permitsin vivohigh-resolution inspection of heart size, shape, and function based on the transparency of the zebrafish embryo. 35
In addition, zebrafish represents a good model for pertur- bation screens due to its small size, fecundity and brief generation time. During embryogenesis the zebrafish embryo does not require a functional cardiovascular system, which enables the study of other- wise lethal cardiac dysfunctions. Large zebrafish screens found.100 mutations affecting heart development and function. 36-39
The frog (Xenopus leavis) has been at the forefront of research in developmental biology for the second half of the last century, which was based on its ease of use for the generation of embryos and sur- gical manipulation. However, its tetraploid genome and long matu- ration time for breeding have been unfavourable for its use as a genetic model. More recently, chemical genetic screens were success- fully performed in Xenopus and open the window to high-throughput screenings. 40
Thus compared with zebrafish it represents a model for investigations of a higher vertebrate system. 41
The fruit fly (Drosophila melanogaster) has been a frequently used model to study the regulation of early heart development. 42,43
As an insect, drosophila is characterized by a simple tube-shaped heart located at the dorsal midline that pumps the haemolymph along its body. The heart, also called dorsal vessel, consists of a single layer of cardiomyocytes, which are surrounded by non-contractile pericar- dial cells. The main advantages of drosophila are the easy use for large genetic screens and for crossing mutants of interest with collections of other mutants out of a large available collection. The cardiac anatomy and less-frequent genetic redundancies made drosophila a widely used model organism for the identification of essential players and regulatory pathways of the heart developmental process. However, this might disqualify drosophila for studies of buf- fering effects, which seem to be essential to understand cardiac altera- tions in human. The mouse (Mus musculus) has been extensively used as a model organism for cardiac developmental malformations in humans. 44
It shares a high degree of homology with humans; but there are fre- quently no direct correlates to human genetic variants such as single nucleotide or copy number variants. A recent study of hetero- zygousNxk2-5knockout mice in systematically varied genetic back- grounds showed the impact of (so far unknown) modifier genes on the penetrance of cardiac malformations. 45
Such model settings are
eligible to systems biology approaches. The generation of a probabil- istic model based on genome-wide sequence variations might even- tually enable to understand the interaction between genetic modifiers andNkx2-5and the phenotypic outcome. Through the
S.R. Sperling270Downloaded from https://academic.oup.com/cardiovascres/article/91/2/269/292858 by guest on 15 August 2023
International Knockout Mouse Consortium a major resource of knockout mouse embryonic stem cells (ES cells) is being generated. 46
To this date, mutated ES cell lines are available for over 15000 protein-coding genes. 47
The laboratory rat (Rattus norvegicus) is the most widely used model organism to decipher how the human organ system functions and is frequently employed for pharmaceutical testing. 48,49
Because of its
size, ease of manipulation and high similarity to the human cardiovas- cular physiology, it remained the preferred choice for respective studies, while the mouse became the leading mammal for experimen- tal genetics in the last decades. Recently, three groups overcame the genetic manipulation limits for rat by developing gene-targeting approaches for gene knockout as well as gene replacement. 50-52
2.1.2 Cell culture and single cell analysis
The heart is built of a mixture of distinct cells from different lineages, which moreover crosstalk to each other. 53
Cardiac fibroblasts rep-
resent?50% of the adult mammalian heart and several types of car- diomyocytes can be distinguished (e.g. atrial vs. ventricular cardiomyocytes). As the heart develops during embryogenesis, cardiac cells migrate a considerable distance and an ever-changing environment influences their fate. An example is neural crest-derived cells contributing to the developing heart. 54,55
One way to study
largely homogenous cell populations according to spatio-temporal changes is the use of cells expressing particular developmental
markers of representative developmental points. For example, cellscould be sorted by fluorescence-activated cell sorting (FACS) for
GFP-positive cells like in Gata4p[5kb]-GFP mouse embryos or using cell layer-specific expression of GFP in transgenic zebrafish. 20,56 Furthermore, cardiomyocytes derived from ES cells or induced pluripotent stem cells (iPSCs) are novel potential sources for systems biology approaches studying differentiation stages of cardiomyocytes.
19,21,57-59
In addition, the mouse atrial-derived cell line HL-1, the rat cell line H9C2 derived from embryonic rat heart tissue and the mouse P19 ES cells are frequently used. 60-62
The contracting HL-1 cardiomyocytes
maintain a gene expression pattern similar to adult cardiomyocytes, whereas H9C2 cells can have both cardiac muscle as well as skeletal muscle properties. The pluripotent P19 embryonal carcinoma cells can differentiate into mesoderm lineages, including cardiomyocytes upon the presence of 1% DMSO. Additionally, a P19Cl6 GFP reporter line, where GFP is expressed under the control of the MLC-2v pro- moter, has been established enabling the quantification of cardiomyocytes. 63
2.1.3 Patients with congenital heart disease
It is clear that genetic manipulations cannot be carried out in man; however, patients with congenital heart disease (CHD) are a unique resource to gain insights into cardiac functional properties and mol- ecular pathways. 64
The genetic alterations underlying the phenotype
of these patients lead to disturbances in the molecular read-out, which can be studied, for example, by gene expression analysis and
Figure 1A framework for systems biology approaches to heart development and congenital heart disease.
Systems biology of heart development and malformation271Downloaded from https://academic.oup.com/cardiovascres/article/91/2/269/292858 by guest on 15 August 2023
potentially provide insights into regulatory relationships. Collections of biomaterial are available through national registries like the CONCOR registry in the Netherlands or the National Registry for
Congenital Heart Defects in Germany.
65,66
Besides the immediate use of patient-material, the generation of patient-specific iPSCs offers a new and very promising way to study human diseases. 67,68
Different groups have already shown the
power of iPSCs for the analysis of congenital arrhythmia and malfor- mation. 69-71
An alternative but more challenging approach is the gen- eration of genetically modified human ES cells using so-called zinc-finger technology to introduce chromosome deletions and trans- locations. 72,73
The advantage of recombinant human ES cell is the
availability of the parental genetically matched ES cell line as a built-in control.
2.2 Cardiac phenotyping approaches
In order to understand biological systems, it is necessary to under- stand the relationship between the genome and the phenotype. The aim would be to monitor the molecular background and the cellular and physiological phenotype at a comparable level of profundity and in a machine-readable manner. In respect to the information gathered, phenotyping approaches can monitor static profiles (e.g. confocal microscopy), or dynamic processes [e.g. echocardiography (ECHO)]. A set of phenotyping approaches relevant for heart devel- opment is described in the following.
2.2.1 Systemic phenotyping of mouse models
Thousands of mouse disease models are now available by the colla- borative effort of the International Mouse Knockout Consortium. 46
The main characterization, archiving, and dissemination of mouse disease models are realized by phenotyping centres (mouse clinics) established in Germany (GSF), France (ICS), and the UK (MRC Harwell and Sanger Institute). These provide large-scale phenotyping platforms and collaborate for the standardization of phenotyping pro- tocols in the EUMODIC consortium (http://www.eumodic.org/). Their current cardiovascular phenotyping consists of blood pressure analysis, electrocardiography, ECHO, and the quantification of bio- markers such as ANP in serum. 25
These phenotyping approaches
are performed on viable adult mice, whereas characterization of structural cardiac or other developmental malformations potentially occurring in embryonic lethal recessive mutations is currently not rea- lized. However, these mouse models would be of particular interest to gain insights into CHD.
2.2.2 Imaging cardiogenesis in live animals
In vivoimaging of the processes that underlie cardiogenesiscell div- ision, migration and differentiation, or haemodynamic parametersis central to understand the structural formation and function of the heart and its molecular background. Since the heart is beating from early stages onward, live time-lapse imaging is particularly challenging. A current lack of technology remains for monitoring of haemo- dynamic parameters like pressure load or mechanical parameter like shear-stress during cardiogenesis. ECHO is one imaging modality extensively used for cardiac assess- ments in clinical settings and has become invaluable for the study of mouse models. Prenatal ultrasound imaging allows longitudinal studies and monitoring of the same animalin vivo. 74
However, the
small size of the foetal mouse heart (,5 mm at birth) makes it tech-
nically challenging. Mouse foetal ECHO can be carried out usingstandard clinical ultrasound systems as well as ultra-high frequency
ultrasound systems; also referred to as ultrasound biomicro- scopes. 75,76
The latter have a two-dimensional spatial resolution that allows monitoring of mouse embryos from embryonic Day 5.5 on. A further method to identify gross cardiac malformations in the mouse embryo is magnetic resonance imaging (MRI). Recent technical advances based on 3D MRI and computational assessment enable the identification of subtle phenotypic differences at E15.5. 29,77
However,
for routine high-throughput application the scan times are very long with 9-36 h and the spatial resolution is limited. High-resolution mag- netic resonance histology and high-resolution episcopic imaging (HREM) start to overcome this limitation. 78,79
Collections of high-
resolution HREM images of mouse and human embryos as well as MRI images of mouse are available online (http://embryoimaging.org, http://mouseatlas.caltech.edu). A routine method to study the transparent zebrafish embryo is live- stream imaging using confocal microscopy. Multiple fluorescent trans- genics and mutants have been established like the Tg(cmlc2:eGFP) and the Tg(flk1:eGFP) zebrafish expressing green fluorescent protein in the cardiac myocytes and endothelial cells, respectively. 80-82
Advanced imaging techniques have been developed which enable the tracking of cardiomyocyte trajectories during cardiac contraction and 3D reconstruction. 26,27
Real-time imaging has further been
applied to monitor the heart tube of the drosophila embryo providing insights into the mechanics of cardiac function. 28
2.2.3 Imaging fixed hearts and monitoring single cells
Classical methods also suitable for high-throughput studies are histo- logical assessment using haematoxylin and eosin staining for morpho- logical inspection,in-situhybridization to visualize transcript expression or immunohistochemistry for protein expression. The gold standard for the visualization of subcellular structures is electron microscopy. A recent interesting technological development is the approach to monitor the interaction between a cell and its physical microenvironment using mechanical tractions exerted by cells in three-dimensional matrices. 83
Further investigations of this method
might eventually be used to gain insights into the role of cellular forces for the developing heart.
2.2.4 Patients with congenital heart disease
In clinical settings, patients with cardiac malformations are studied using ECHO, MRI, or cardiac catheterization. In 2005 the International Pediatric and Congenital Cardiac Code (IPCCC, www.ipccc.net) was launched, which enables a detailed description of anatomic and haemodynamic features in CHD in human and provides a unique source for a standardized phenotype description. 84
2.2.5 Computational coding of cardiac phenotypes
Computational coding of phenotypes and machine-readable represen- tation of biological models is essential for any systems biology approach. In contrast to the computational coding system of CHD in human, a defined ontology describing the precise cardiac anatomy, morphology, and haemodynamic features of model organ- isms is currently missing. The establishment of such phenotype ontol- ogy remains, therefore, a central goal for respective systems biology studies.
S.R. Sperling272Downloaded from https://academic.oup.com/cardiovascres/article/91/2/269/292858 by guest on 15 August 2023
2.3 Biotechniques for systems biology
Techniques used in systems biology approaches can in general be grouped into techniques measuring molecular states and techniques measuring molecular interactions. A number of technical develop- ments have been used as the basis for systems level studies building a ground floor for systems biology. One major impact emanated from the microarray technology to analyse gene expression profiles (DNA microarray) and as a general application to measure nucleotide sequence or protein abundances derived by a panel of different primary selection and enrichment processes.
2.3.1 Genome, epigenome, and transcriptome analysis
Genomic sequences can be enriched based on antibody-specific selection using chromatin-immunoprecipitation (ChIP) to measure transcription factor binding events (ChIP-chip) or occurrence of histone modifications, or the presence of DNA methylation (MeDIP- chip). 85,86
A further selection method for DNA methylation is based on the hypersensitivity to DNaseI cleavage (DNase-chip). 87
An elegant way to overcome the need of factor-specific antibodies is DamID chromatin profiling, here the bacterial Dam-methylase is fused to a protein of interest and subsequently marks its genomic- binding site. 88,89
Other applications are the analysis of sequence vari- ations by genotyping microarrays, 90
copy number variations (Array-CGH) or RNA profiling (mRNA, microRNA). 91
So far, these
methods have been the main basis for systems biology studies of heart development and its alterations. Nowadays, microarrays are replaced more and more by next generation sequencing (NGS) tech- nologies. The currently most frequently used NGS platforms are 454 (Roche Diagnostics), Solexa (Illumina), and SOLiD (Applied Biosys- tems). 92
Each of these technologies has their advantages and disadvan- tages. Four hundred and fifty-four sequencing is based on pyrosequencing and enables sequencing of roughly 400-600 Mb of DNA per 10-h run with an average read length of 400 bases on the Genome Sequencer FLX. The long read lengths are the main advan- tage of this technique, whereas it is also the most expensive platform.
The Solexa Genome Analyzer IIx system based on
sequencing-by-synthesis process requires?2 days to generate read lengths of 35-150 bases and an overall sequence output of 10-
12 Gb per run. The SOLiD (Supported Oligonucleotide Ligation
and Detection) platform is also a short-read (35-75 bases) sequen- cing technology based on ligation. The 5500 SOLiD system generates up to 10-15 Gb per day. The first single-molecule sequencing plat- form (HeliScope) is available from Helicos BioSciences (http://www. helicosbio.com) with an output of 1 Gb/day and read lengths of up to 45-50 bases. 23
2.3.2 Proteome and metabolome analysis
Mass spectrometry (MS) and nuclear magnetic resonance spec- troscopy (NMR) are the core technologies for proteome and meta- bolome studies. 93,94
The majority of approaches is based on the
analysis of peptides, which are frequently generated by enzymatic digestion of proteins. The selection and enrichment of the fraction of proteins/peptides of interest is a key issue for MS analysis. Selection strategies can be applied for subcellular fractioning (e.g. membrane enrichment, nucleus precipitation, or mitochondria separation), for a particular protein and its interaction partners (e.g. coimmunopreci- pitation) or proteins harbouring particular modifications (e.g. phos-
phorylation). A particular interesting approach is SILAC, whichrelies on the incorporation of isotopically labelled amino acids into
proteins during their synthesis. 95
This technique can be applied to
cell culture studies and more recently also to study mouse and dro- sophila models, which opens a novel way to study proteome differ- ences in respective model systems. 96-98
NMR is a common method in metabolomics and in contrast to MS does not require analyte separation. It can provide detailed infor- mation on the molecular structure of compounds found in complex mixtures, such as biofluids and cell and tissue extracts, which can be e.g. analysed through the use of 1
H NMR sprectroscopy.
99
Long-standing methods for the analysis of protein-protein inter- actions are the yeast-two-hybrid (Y2H) and the mammalian-two- hybrid (M2H) systems. In these systems, a read-out is achieved via the protein-protein interaction of the two proteins to be tested, which are expressed as hybrid fusion proteins. Automated and high- throughput Y2H experiments identified over 3000 interactions among 1705 human proteins resulting in a large and highly connected network and a M2H study provided a map of physical interactions among 762 human and 877 mouse DNA-binding transcription factors.
100,101
Besides the two-hybrid systems, peptide microarrays have been employed to study protein-protein interactions; however, their implementation has been much slower compared with DNA arrays because of technical challenges such as high- throughput synthesis of peptides at a low cost. 102
2.3.3 Techniques to induce perturbations
In vivogene targeting techniques are essential to enable the study of cause-effect relationships. 103
Gene targeting in ES cells is widely
used to generate designed mouse mutants. Knockout mice harbouring a null allele in their germline represent essential genetic models for the study of inherited disease, whereas conditional gene inactivation is extensively used for the study of gene function in adult mice as well as in specific cell types. 104
The latter relies on the DNA recom-
binase Cre and its recognition (loxP) sites. In the last decade, a large collection of Cre transgenic lines has been generated and can be used in a combinatorial manner to attain gene inactivation in many different cell types or starting at a defined embryonic stage. This approach is particularly useful for models targeting cardiac essential genes, which otherwise might lead to very early embryonic lethal pheno- types. The application of CreER(T2) transgenic mice enables the inac- tivation of floxed alleles in adult mice upon administration of tamoxifen. A widely used approach for gene knockdown is RNA inter- ference (RNAi) and large genome-scale RNAi high-throughput screens have been carried out in both drosophila and mammalian cul- tured cells. 105
In zebrafish, Morpholino oligonucleotides (MOs) are the most common anti-sense knockdown" technique. MOs bind RNA, thereby facilitating steric hindrance of proper transcript proces- sing or translation. 106
Small molecules and chemical screens have played an important role in delineating molecular pathways involved in embryonic develop- ment and disease pathology. In contrast to directedin vivogene target- ing approaches, chemical screens enable the discovery of gene functions in an unbiased manner. In mice, chemical mutagenesis induced byN-ethyl-N-nitrosourea (ENU) provides a unique resource for mutants and displays a range of mutant effects from complete or partial loss of function to exaggerated function.
107,108
A furthermore
well-suited model for use in chemical screens is zebrafish, where small molecules are typically added to the aquatic environment in which
Systems biology of heart development and malformation273Downloaded from https://academic.oup.com/cardiovascres/article/91/2/269/292858 by guest on 15 August 2023
they live, allowing absorption into the fish without the need for inva- sive and time-consuming injection.
38,109,110
Besides gene targeting approaches, environmental alterations gov- erning perturbations of molecular networks allow the analysis of gene-environment interactions, which might play a particular role in the development of CHD with incomplete penetrance. 32
2.4 Computational analysis
Computational approaches have mainly universal applicability, which is in contrast to the specifications of data gathering (models system, molecular level, organ focus, etc.). Recent developments of compu- tational analysis strategies, modelling approaches, and software packages are reviewed elsewhere.
4,111,112
A particular aspect for analysing a developmental process such as cardiogenesis is the fact that information flow may use different pathways at different developmental time points. Thus, tools that can model dynamic regulatory networks phasing over time are important for the study of heart development. 113
For example,
binding of a particular transcription factor to DNA may only modu- late gene expression in concert with an epigenetic modification, which occurs only at a specific developmental window, or a defined outcome of gene expression may depend on the impact of a panel of different factors acting in concert on this regulatory region. 114
Therefore, nodes in a transcription network do not necessarily represent essential points at a particular developmental stage, but might rather be elements enabling fine-tuning of transcrip- tional and thus developmental responses to environmental signals. Computational modelling might provide the opportunity to define the genetic and epigenetic impact in the context of variable internal and external, environmental, and stochastic influences.
3. Knowledge gained by recent
studies In the last decade, deep insight was gained on how a number of cardiac-restricted transcription factors act in concert to govern cardi- ogenesis.
115-117
However, at latest with the discovery of the impact of chromatin remodelling factors (e.g. Baf60 or Baf45c/DPF3) or histone-modifying enzyme (e.g. Jmjd6) it is obvious that the molecular network underlying heart development and CHD is far more complex.
118-120
A challenge is bringing together the many uncovered molecular components driving heart development and eventually to establish computational models with predictive power. This is even complicated by the unknown level of the environmental and stochas- tic impact as well as the nature and capacity of buffering mechanism of the system (Figure2). Besides the map of the physical components and interactions driving cardiogenesis, insights have to be gained on how the flow of information propagates and responses to perturbations. Given an example, maternal high-fat diet of Cited2 haploinsufficient mice increases the risk (penetrance) of CHD in their offspring, 32
if this holds through in human is an open question. 121
The reduced
expression of Pitx2c in these mice might be a key driver for the obser- vation, but we currently lack understanding of the mechanism behind. It is unknown how the observed maternal hyperglycaemia is trans- duced to the genome leading to expression changes of respective genes and, moreover, which cascades are initiated by it resulting in the phenotypic endpoint. Therefore, critical mass of data need to be gathered in appropriate model systems, and computational approaches must utilize the biological information at the different levels by integrating the heterogeneous data and finally enable predic- tion of molecular and phenotypic outcome induced by perturbations. Insights into cardiogenesis are primarily generated by the study of embryonic models and with constraints using cell-culture systems.
Figure 2Simplified overview of the aetiology of congenital heart disease (CHD) reflecting multifactorial impacts on heart development. Biological
networks (orange) mediate interactions between particular DNA sequences (blue; e.g. genes, miRNAs), epigenetic modifications (grey; DNA methyl-
ation, histone modification), and distinct phenotypes (red). The aetiology of CHD ranges from simple relationships between single genes and a par-
ticular type of CHD up to an underlying complex network influenced by multiple genes, epigenetic, environmental influences, (green) and potentially
stochastic events (purple). Environmental influences can be external" as well as internal" to the system. Congenital heart diseases are depicted asin
part overlapping phenotypes, which is one characteristic feature of cardiac alterations and reflects the modularity of heart development.
S.R. Sperling274Downloaded from https://academic.oup.com/cardiovascres/article/91/2/269/292858 by guest on 15 August 2023
A cardiovascular development signalling network constructed based on gene expression profiles of ES cells undergoing guided differen- tiation to cardiomyocytes showed that selected nodes orchestrate coordinated recruitment of specialized ontological classes to secure a developmental theme. 122
Integrins and WNT/b-catenin were the
most significant cascades identified. Their relevance was further shown by a proteomic-based study of mouse embryos developing congenital heart defects after exposure to high glucose and corre- spondingly increased protein levels could be observed in the amniotic fluid of human foetuses with CHD. 123
A further study based on
protein interaction networks showed that distinct clusters of interact- ing proteins (so-called functional modules") underlie heart develop- ment characterized by the development of defined anatomical substructures. The study suggests that higher order functional net- works are achieved by the integration of discrete protein complexes in the way that evolutionary younger anatomical structures are poten- tially parts of networks already used in more ancient structures.
124,125
Here, 255 proteins grouped into 19 morphological subgroups where used as an indicator for spatio-temporal function of individual genes. Thus, the work points to the concept of combinatorial regulation at the protein level, which was confirmed recently in a study investi- gating the transcription factors Gata4, Mef2a, Nkx2.5, and Srf. 114
These factors showed a high degree of combinatorial regulation andmoreover, can partially compensate each other"s function (Figure3).
This has direct implication for our understanding of the molecular background of CHD as it might explain incomplete penetrance and disease variability depending on the degree of functional alterations of discrete factors or in combination with secondary insults (e.g. environmental effects). Besides focusing on one molecular level such as the genome, transcriptome, or proteome independently, it is essential to interrogate different levels in order to understand the full biological architecture. It was shown that the functional read-out generated by distinct cardiac transcription factors such as Srf and Gata4 can be modulated by complementary histone acety- lation and that regulatory circuits between the transcription factor Srf and microRNAs might contribute a high degree of indirectly affected targets genes in the situation of reduced function. 114
Further insights into regulatory cardiac transcription networks have been gained by interrogating gene expression in hearts of CHD patients and the anatomical, morphological, and haemodynamic fea- tures of these malformed hearts. 64
Networks of correlated gene
groups could be identified pointing once more to the modular archi- tecture underlying heart development. In addition to the immediate study of heart development, the analy- sis of cardiac disease harbouring remodelling features can also provide insights into network modules. Dilated cardiomyopathy is one such
Figure 3Regulation of the cardiac transcriptome by combinatorial binding of the transcription factors Gata4 (green), Mef2a (red), Nkx2.5 (dark
blue), and Srf (light blue). 135
Shown are in total 1671 genes, which are targeted by at least one factor. All four factors share 91 target genes and sets of
three and two factors share 121 and 286 genes, respectively. The combinatorial binding by these four key cardiac transcription factors is in line with
their combinatorial regulation of shared down-stream targets, which is moreover interrogated by a number of indirect regulatory effects through
microRNAs as well as epigenetic marks such as histone modifications. 135
This illustrates the complex genetic, epigenetic, and environmental back- ground underlying heart development and congenital heart disease.
Systems biology of heart development and malformation275Downloaded from https://academic.oup.com/cardiovascres/article/91/2/269/292858 by guest on 15 August 2023
exemplary cardiac disorder. A recent study of dilated cardiomyopathy analysed protein-protein interaction networks in combination with condition-specific co-expression information.
64,126
Two dynamic
modules, consisting of proteins essential for muscle contraction and organ morphogenesis, respectively, could be identified. Even though the importance of edges and nodes might differ during normal cardi- ogenesis, the general network properties identified should be con- sidered with the disease state as a perturbation model. In a further high-throughput proteome study with integrated network biology, the consequences of Kir6.2 KATP channel pore deletion were ana- lysed in respective knockout mice showing cardiomyopathic predispo- sition. 127
Based on the integration of proteome and gene expression data, a KATP channel-associated subproteome could be identified and further assessment of this network showed the primary impact of the Kir6.2 KATP channel on metabolic pathways associated with cardio- vascular disease.
4. Limitations of systems biology
Modelling of pieces that are interlinked in a way we do not understand yet is challenging and further complicated by the need to separate background noise from signal, which is a common problem of high- throughput data. The analysis of high-throughput data is based on the application of suitable statistical models, which estimate the signifi- cance of observed correlations and other dependencies. Thus, it is most crucial to select appropriate models and to carefully deal with the influence of noise and the high number of performed tests, e.g. by suitable correction for multiple testing. Although systems biology approaches is highly suggestive to derive new hypotheses, well- defined gene/protein-focused or pathway-focused experiments are essential to complement it and define the extent to which insights gained at a global scale hold true for specific segments of the system.
5. Concluding remarks and future
perspective The heart is the first organ fully functioning during development, and CHDs are the most common birth defect in human. Despite the heart being one of the central organs, we still have limited understanding about the molecular background driving heart development and its interferences leading to the burden caused by alterations of this process. The heart develops based on evolutionary conserved sequences of functional modules. Over the last decades important key regulators have been identified and insights have been gained by the study of animal models, as well as patients with CHD. Cardiac malformations represent a broad panel of in part overlapping pheno- types, potentially reflecting the modular background of cardiogenesis. However, the biological network modifying the impact of key regula- tors is still widely a black box. One important lesson learned from the study of animal models, as well as patients with complex CHD is that our primary hope that one gene would simply refer to one phenotype is not fulfilled. Even one particular mutation can be associated with a panel of different cardiac malformations and the majority of CHD is not following Mendelian inheritance. Nevertheless, it is undoubtful that there is a clear genetic impact.
128,129
We currently enter a
novel era of research, which is characterized by fast evolving techno- logical advances enabling the generation, study and interpretation of more and more complex data. It is highly suggestive that this will
allow the study of heart development in a systems biology mannerthat provides insights into the biological network influenced by
genetic, epigenetic and environmental factors, and stochastic events. Two strategies can be envisaged: approaches utilizing already gener- ated data (e.g. gene expression profiles and transcription factor-DNA binding)
89,130-132
and the set-up of interdisciplinary projects where data generation (in particular perturbations) is driven by the input needs of modelling. The extraordinary conservation of the heart developmental process and its genes between human and model organisms enables the integration of data from different species.
133,134
Hopefully, we can one day understand the causative impacts leading to the majority of CHD at some point, and thereof push forward the development of novel therapeutic and, even more important, preventive options such as e.g. folic acid treatment in place today.
Acknowledgements
I am grateful to Markus Schu¨ler and Rainer Dunkel for preparation of graphics and thank Marcel Grunert, Cornelia Dorn and Markus Schu¨ler for discussion and review of the manuscript. I apologize to those who have made contributions to our understanding of this research area and are not cited in this review.
Conflict of interest:none declared.
Funding
This work was supported by the European Community"s Seven Frame- work Program contract (CardioGeNet") 2009-223463.
References
1. Hodgkin AL, Huxley AF. A quantitative description of membrane current and its
application to conduction and excitation in nerve.J Physiol (Lond)1952;117:500-544.
2. Noble D. A modification of the Hodgkin-Huxley equations applicable to Purkinje
fibre action and pace-maker potentials.J Physiol1962;160:317-352.
3. Macilwain C. Systems biology: evolving into the mainstream.Cell2011;144:839-841.
4. Chuang HY, Hofree M, Ideker T. A decade of systems biology.Ann Rev Cell Dev Biol
2010;26:721-744.
5. Deichmann U. Early 20th-century research at the interfaces of genetics, develop-
ment, and evolution: reflections on progress and dead ends.Dev Biol2011 Mar
21. [Epub ahead of print]
6. Kohl P, Crampin EJ, Quinn TA, Noble D. Systems biology: an approach.Clin Pharma-
col Ther2010;88:25-33.
7. Adams KF. Systems biology and heart failure: concepts, methods, and potential
research applications.Heart Fail Rev2010;15:371-398.
8. Kohl P, Noble D. Systems biology and the virtual physiological human.Mol Syst Biol
2009;5:292.
9. Noble D. Systems biology and the heart.BioSystems2006;83:75-80.
10. Jothi R, Balaji S, Wuster A, Grochow JA, Gsponer J, Przytycka TMet al. Genomic
analysis reveals a tight link between transcription factor dynamics and regulatory network architecture.Mol Syst Biol2009;5:294.
11. Davidson EH, Rast JP, Oliveri P, Ransick A, Calestani C, Yuh C-Het al. A genomic
regulatory network for development.Science2002;295:1669-1678.
12. de la Fuente A. From differential expression" to differential networking"identifi-
cation of dysfunctional regulatory networks in diseases.Trends Genet2010;26:
326-333.
13. Artyomov MN, Meissner A, Chakraborty AK. A model for genetic and epigenetic
regulatory networks identifies rare pathways for transcription factor induced pluri- potency.PLoS Comput Biol2010;6:e1000785.
14. Hyduke DR, Palsson BO. Towards genome-scale signalling-network reconstructions.
Nat Rev Genet2010;11:297-307.
15. Kim TY, Kim HU, Lee SY. Data integration and analysis of biological networks.Curr
Opin Biotechnol2010;21:78-84.
16. Stainier DY, Fishman MC. The zebrafish as a model system to study cardiovascular
development.Trends in cardiovascular medicine1994;4:207-212.
17. Vuillemin M, Pexieder T. Normal stages of cardiac organogenesis in the mouse: II.
Development of the internal relief of the heart.Am J Anat1989;
184:114-128.
18. Vuillemin M, Pexieder T. Normal stages of cardiac organogenesis in the mouse: I.
Development of the external shape of the heart.Am J Anat1989;184:101-113.
19. Beqqali A, van Eldik W, Mummery C, Passier R. Human stem cells as a model for
cardiac differentiation and disease.Cell Mol Life Sci2009;66:800-813.
S.R. Sperling276Downloaded from https://academic.oup.com/cardiovascres/article/91/2/269/292858 by guest on 15 August 2023
20. Hadjantonakis AK, Nagy A. FACS for the isolation of individual cells from transgenic
mice harboring a fluorescent protein reporter.Genesis2000;27:95-98.
21. Ieda M, Fu J-D, Delgado-Olguin P, Vedantham V, Hayashi Y, Bruneau BGet al. Direct
reprogramming of fibroblasts into functional cardiomyocytes by defined factors.Cell
2010;142:375-386.
22. Navin N, Kendall J, Troge J, Andrews P, Rodgers L, McIndoo Jet al. Tumour evol-
ution inferred by single-cell sequencing.Nature2011;472:90-94.
23. Schadt EE, Turner S, Kasarskis A. A window into third-generation sequencing.Hum
Mol Genet2010;19:R227-R240.
24. Musunuru K, Domian IJ, Chien KR. Stem cell models of cardiac development and
disease.Ann Rev Cell Dev Biol2010;26:667-687.
25. Gailus-Durner V, Fuchs H, Adler T, Aguilar Pimentel A, Becker L, Bolle Iet al. Sys-
temic first-line phenotyping.Methods Mol Biol2009;530:463-509.
26. Ohn J, Tsai H-J, Liebling M. Joint dynamic imaging of morphogenesis and function in
the developing heart.Organogenesis2009;5:166-173.
27. Scherz PJ, Huisken J, Sahai-Hernandez P, Stainier DYR. High-speed imaging of devel-
oping heart valves reveals interplay of morphogenesis and function.Development
2008;135:1179-1187.
28. Wu M, Sato TN. On the mechanics of cardiac function of Drosophila embryo.PLoS
ONE2008;3:e4045.
29. Zamyadi M, Baghdadi L, Lerch JP, Bhattacharya S, Schneider JE, Henkelman RMet al.
Mouse embryonic phenotyping by morphometric analysis of MR images.Physiol
Genomics2010;42A:89-95.
30. Kaynak B, von Heydebreck A, Mebus S, Seelow D, Hennig S, Vogel Jet al. Genome-
wide array analysis of normal and malformed human hearts.Circulation2003;107:
2467-2474.
31. Bazzano LA. Folic acid supplementation and cardiovascular disease: the state of the
art.Am J Med Sci2009;338:48-49.
32. Bentham J, Michell AC, Lockstone H, Andrew D, Schneider JE, Brown NAet al.
Maternal high-fat diet interacts with embryonic Cited2 genotype to reduce Pitx2c expression and enhance penetrance of left-right patterning defects.Hum Mol
Genet2010;19:3394-3401.
33. Cittadini A, Mantzoros CS, Hampton TG, Travers KE, Katz SE, Morgan JPet al. Car-
diovascular abnormalities in transgenic mice with reduced brown fat: an animal model of human obesity.Circulation1999;100:2177-2183.
34. Bassingthwaighte J, Hunter P, Noble D. The cardiac physiome: perspectives for the
future.Exp Physiol2009;94:597-605.
35. Schoenebeck JJ, Yelon D. Illuminating cardiac development: advances in imaging add
new dimensions to the utility of zebrafish genetics.Semin Cell Dev Biol2007;18:
27-35.
36. Chen JN, Haffter P, Odenthal J, Vogelsang E, Brand M, van Eeden FJet al. Mutations
affecting the cardiovascular system and other internal organs in zebrafish.Develop- ment1996;123:293-302.
37. Chi NC, Shaw RM, Jungblut B, Huisken J, Ferrer T, Arnaout Ret al. Genetic and
physiologic dissection of the vertebrate cardiac conduction system.PLoS Biol2008;
6:e109.
38. Molina G, Vogt A, Bakan A, Dai W, Queiroz de Oliveira P, Znosko Wet al. Zebrafish
chemical screening reveals an inhibitor of Dusp6 that expands cardiac cell lineages.
Nat Chem Biol2009;5:680-687.
39. Stainier DY, Fouquet B, Chen JN, Warren KS, Weinstein BM, Meiler SEet al.
Mutations affecting the formation and function of the cardiovascular system in the zebrafish embryo.Development1996;123:285-292.
40. Tomlinson ML, Field RA, Wheeler GN. Xenopus as a model organism in develop-
mental chemical genetic screens.Mol Biosyst2005;1:223-228.
41. Gilchrist MJ, Christensen MB, Bronchain O, Brunet F, Chesneau A, Fenger Uet al.
Database of queryable gene expression patterns for Xenopus.Dev Dyn2009;238:
1379-1388.
42. Reim I, Frasch M. Genetic and genomic dissection of cardiogenesis in the Drosophila
model.Pediatr Cardiol2010;31:325-334.
43. Tao Y, Schulz RA. Heart development in Drosophila.Semin Cell Dev Biol2007;18:
3-15.
44. Blake JA, Bult CJ, Kadin JA, Richardson JE, Eppig JT. Group tMGD. The mouse
genome database (MGD): premier model organism resource for mammalian geno- mics and genetics.Nucleic Acids Res2011;39:D842-848.
45. Winston JB, Erlich JM, Green CA, Aluko A, Kaiser KA, Takematsu Met al. Hetero-
geneity of genetic modifiers ensures normal cardiac development.Circulation2010;
121:1313-1321.
46. Consortium IMK, Collins FS, Rossant J, Wurst W. A mouse for all reasons.Cell2007;
128:9-13.
47. Ringwald M, Iyer V, Mason JC, Stone KR, Tadepally HD, Kadin JAet al. The IKMC
web portal: a central point of entry to data and resources from the International Knockout Mouse Consortium.Nucleic Acids Res2011;39:D849-855.
48. Aitman TJ, Critser JK, Cuppen E, Dominiczak A, Fernandez-Suarez XM, Flint Jet al
. Progress and prospects in rat genetics: a community view.Nat Genet2008;40:
516-522.
49. Gill TJ, Smith GJ, Wissler RW, Kunz HW. The rat as an experimental animal.Science
1989;245:269-276.50. Izsva
´k Z, Fro¨hlich J, Grabundzija I, Shirley JR, Powell HM, Chapman KMet al. Gen- erating knockout rats by transposon mutagenesis in spermatogonial stem cells.Nat
Methods2010;7:443-445.
51. Tong C, Li P, Wu NL, Yan Y, Ying Q-L. Production of p53 gene knockout rats by
homologous recombination in embryonic stem cells.Nature2010;467:211-213.
52. Geurts AM, Cost GJ, Freyvert Y, Zeitler B, Miller JC, Choi VMet al. Knockout rats
via embryo microinjection of zinc-finger nucleases.Science2009;325:433.
53. Ieda M, Tsuchihashi T, Ivey KN, Ross RS, Hong TT, Shaw RMet al. Cardiac fibro-
blasts regulate myocardial proliferation through beta1 integrin signaling.Dev Cell
2009;16:233-244.
54. Creazzo TL, Godt RE, Leatherbury L, Conway SJ, Kirby ML. Role of cardiac neural
crest cells in cardiovascular development.Ann Rev Physiol1998;60:267-286.
55. Theveneau E, Mayor R. Collective cell migration of the cephalic neural crest: the art
of integrating information.Genesis2011;49:164-176.
56. Pilon N, Raiwet D, Viger RS, Silversides DW. Novel pre- and post-gastrulation
expression of Gata4 within cells of the inner cell mass and migratory neural crest cells.Dev Dyn2008;237:1133-1143.
57. Synnergren J, Akesson K, Dahlenborg K, Vidarsson H, Ame
´en C, Steel Det al. Mol-
ecular signature of cardiomyocyte clusters derived from human embryonic stem cells.Stem Cells2008;26:1831-1840.
58. Xu XQ, Soo SY, Sun W, Zweigerdt R. Global expression profile of highly enriched
cardiomyocytes derived from human embryonic stem cells.Stem Cells2009;27:
2163-2174.
59. Yang L, Soonpaa MH, Adler ED, Roepke TK, Kattman SJ, Kennedy Met al. Human
cardiovascular progenitor cells develop from a KDR+embryonic-stem-cell-derived population.Nature2008;453:524-528.
60. Claycomb WC, Lanson NA, Stallworth BS, Egeland DB, Delcarpio JB, Bahinski A
et al. HL-1 cells: a cardiac muscle cell line that contracts and retains phenotypic characteristics of the adult cardiomyocyte.Proc Natl Acad Sci USA1998;95 :
2979-2984.
61. Kimes BW, Brandt BL. Properties of a clonal muscle cell line from rat heart.Exp Cell
Res1976;98:367-381.
62. McBurney MW, Jones-Villeneuve EM, Edwards MK, Anderson PJ. Control of muscle
and neuronal differentiation in a cultured embryonal carcinoma cell line.Nature
1982;299:165-167.
63. Moore JC, Spijker R, Martens AC, de Boer T, Rook MB, van der Heyden MAet al.A
P19Cl6 GFP reporter line to quantify cardiomyocyte differentiation of stem cells.Int
J Dev Biol2004;48:47-55.
64. Toenjes M, Schueler M, Hammer S, Pape UJ, Fischer JJ, Berger Fet al. Prediction of
cardiac transcription networks based on molecular data and complex clinical pheno- types.Mol Biosyst2008;4:589-598.
65. van der Velde ET, Vriend JW, Mannens MM, Uiterwaal CS, Brand R, Mulder BJ.
CONCOR, an initiative towards a national registry and DNA-bank of patients with congenital heart disease in the Netherlands: rationale, design, and first results.Eur J Epidemiol2005;20:549-557.
66. Goebel JW, Pickardt T, Bedau M, Fuchs M, Lenk C, Paster Iet al. Legal and ethical
consequences of international biobanking from a national perspective: the German BMB-EUCoop project.Eur J Hum Genet2010;18:522-525.
67. Park IH, Arora N, Huo H, Maherali N, Ahfeldt T, Shimamura Aet al. Disease-specific
induced pluripotent stem cells.Cell2008;134:877-886.
68. Domian IJ, Chiravuri M, van der Meer P, Feinberg AW, Shi X, Shao Yet al. Gener-
ation of functional ventricular heart muscle from mouse ventricular progenitor cells.
Science2009;326:426-429.
69. Itzhaki I, Maizels L, Huber I, Zwi-Dantsis L, Caspi O, Winterstern Aet al. Modelling
the long QT syndrome with induced pluripotent stem cells.Nature2011;471:
225-229.
70. Carvajal-Vergara X, Sevilla A, D"Souza SL, Ang YS, Schaniel C, Lee DFet al. Patient-
specific induced pluripotent stem-cell-derived models of LEOPARD syndrome.
Nature2010;465:808-812.
71. Moretti A, Bellin M, Welling A, Jung CB, Lam JT, Bott-Flugel Let al. Patient-specific
induced pluripotent stem-cell models for long-QT syndrome.New Eng J Med2010;
363:1397-1409.
72. Porteus MH, Carroll D. Gene targeting using zinc finger nucleases.Nat Biotechnol
2005;23:967-973.
73. Zou J, Maeder ML, Mali P, Pruett-Miller SM, Thibodeau-Beganny S, Chou BKet al.
Gene targeting of a disease-related gene in human induced pluripotent stem and embryonic stem cells.Cell Stem Cell
2009;5:97-110.
74. Keller BB, MacLennan MJ, Tinney JP, Yoshigi M.In vivoassessment of embryonic car-
diovascular dimensions and function in day-10.5 to -14.5 mouse embryos.Circ Res
1996;79:247-255.
75. Phoon CKL. Imaging tools for the developmental biologist: ultrasound biomicro-
scopy of mouse embryonic development.Pediatr Res2006;60:14-21.
76. Yu Q, Leatherbury L, Tian X, Lo CW. Cardiovascular assessment of fetal mice by in
utero echocardiography.Ultrasound Med Biol2008;34:741-752.
77. Zouagui T, Chereul E, Janier M, Odet C. 3D MRI heart segmentation of mouse
embryos.Comput Biol Med2010;40:64-74.
78. Petiet AE, Kaufman MH, Goddeeris MM, Brandenburg J, Elmore SA, Johnson GA.
High-resolution magnetic resonance histology of the embryonic and neonatal
Systems biology of heart development and malformation277Downloaded from https://academic.oup.com/cardiovascres/article/91/2/269/292858 by guest on 15 August 2023
mouse: a 4D atlas and morphologic database.Proc Natl Acad Sci USA2008;105:
12331-12336.
79. Pieles G, Geyer SH, Szumska D, Schneider J, Neubauer S, Clarke Ket al.
microMRI-HREM pipeline for high-throughput, high-resolution phenotyping of murine embryos.J Anat2007;211:132-137.
80. Beis D, Bartman T, Jin S-W, Scott IC, D"Amico LA, Ober EAet al. Genetic and cel-
lular analyses of zebrafish atrioventricular cushion and valve development.Develop- ment2005;132:4193-4204.
81. Huang C-J, Tu C-T, Hsiao C-D, Hsieh F-J, Tsai H-J. Germ-line transmission of a
myocardium-specific GFP transgene reveals critical regulatory elements in the cardiac myosin light chain 2 promoter of zebrafish.Dev Dyn2003;228:30-40.
82. Motoike T, Loughna S, Perens E, Roman BL, Liao W, Chau TCet al. Universal GFP
reporter for the study of vascular development.Genesis2000;28:75-81.
83. Legant WR, Miller JS, Blakely BL, Cohen DM, Genin GM, Chen CS. Measurement of
mechanical tractions exerted by cells in three-dimensional matrices.Nat Methods
2010;7:969-971.
84. Jacobs JP, Mavroudis C, Jacobs ML, Maruszewski B, Tchervenkov CI,
Lacour-Gayet FGet al. Nomenclature and databasesthe past, the present, and the future: a primer for the congenital heart surgeon.Pediatr Cardiol2007;28:
105-115.
85. Weng YI, Huang TH, Yan PS. Methylated DNA immunoprecipitation and
microarray-based analysis: detection of DNA methylation in breast cancer cell lines.Methods Mol Biol2009;590:165-176.
86. Horak CE, Snyder M. ChIP-chip: a genomic approach for identifying transcription
factor binding sites.Methods Enzymol2002;350:469-483.
87. Shibata Y, Crawford GE. Mapping regulatory elements by DNaseI hypersensitivity
chip (DNase-Chip).Methods Mol Biol2009;556:177-190.
88. van Steensel B, Henikoff S. Identification ofin vivoDNA targets of chromatin pro-
teins using tethered dam methyltransferase.Nat Biotechnol2000;18:424-428.
89. Vogel MJ, Peric-Hupkes D, van Steensel B. Detection ofin vivoprotein-DNA inter-
actions using DamID in mammalian cells.Nat Protoc2007;2:1467-1478.
90. Szelinger S, Pearson JV, Craig DW. Microarray-based genome-wide association
studies using pooled DNA.Methods Mol Biol2011;700:49-60.
91. Solinas-Toldo S, Lampel S, Stilgenbauer S, Nickolenko J, Benner A, Dohner Het al.
Matrix-based comparative genomic hybridization: biochips to screen for genomic imbalances.Genes Chromosomes Cancer1997;
20:399-407.
92. Voelkerding KV, Dames SA, Durtschi JD. Next-generation sequencing: from basic
research to diagnostics.Clin Chem2009;55:641-658.
93. Elliott MH, Smith DS, Parker CE, Borchers C. Current trends in quantitative proteo-
mics.J Mass Spectrom2009;44:1637-1660.
94. Turer AT, Stevens RD, Bain JR, Muehlbauer MJ, van der Westhuizen J, Mathew JP
et al. Metabolomic profiling reveals distinct patterns of myocardial substrate use in humans with coronary artery disease or left ventricular dysfunction during surgical ischemia/reperfusion.Circulation2009;119:1736-1746.
95. Ong SE, Blagoev B, Kratchmarova I, Kristensen DB, Steen H, Pandey Aet al. Stable
isotope labeling by amino acids in cell culture, SILAC, as a simple and accurate approach to expression proteomics.Mol Cell Proteomics2002;1:376-386.
96. Kru¨ger M, Moser M, Ussar S, Thievessen I, Luber CA, Forner Fet al. SILAC mouse
for quantitative proteomics uncovers kindlin-3 as an essential factor for red blood cell function.Cell2008;134:353-364.
97. Selbach M, Schwanha¨usser B, Thierfelder N, Fang Z, Khanin R, Rajewsky N. Wide-
spread changes in protein synthesis induced by microRNAs.Nature2008;455:
58-63.
98. Sury MD, Chen JX, Selbach M. The SILAC fly allows for accurate protein quantifi-
cationin vivo.Mol Cell Proteomics2010;9:2173-2183.
99. Beckonert O, Keun HC, Ebbels TM, Bundy J, Holmes E, Lindon JCet al. Metabolic
profiling, metabolomic and metabonomic procedures for NMR spectroscopy of urine, plasma, serum and tissue extracts.Nat Protoc2007;2:2692-2703.
100. Stelzl U, Worm U, Lalowski M, Haenig C, Brembeck FH, Goehler Het al. A human
protein-protein interaction network: a resource for annotating the proteome.Cell
2005;122:957-968.
101. Ravasi T, Suzuki H, Cannistraci CV, Katayama S, Bajic VB, Tan Ket al. An atlas of
combinatorial transcriptional regulation in mouse and man.Cell2010;140:744-752.
102. Henderson G, Bradley M. Functional peptide arrays for high-throughput chemical
biology based applications.Curr Opin Biotechnol2007;18:326-330.
103. Hardy S, Legagneux V, Audic Y, Paillard L. Reverse genetics in eukaryotes.Biol Cell
2010;102:561-580.
104. Friedel RH, Wurst W, Wefers B, Kuhn R. Generating conditional knockout mice.
Methods Mol Biol2011;693:205-231.
105. Mohr S, Bakal C, Perrimon N. Genomic screening with RNAi: results and challenges.
Ann Rev Biochem2010;
79:37-64.
106. Bill BR, Petzold AM, Clark KJ, Schimmenti LA, Ekker SC. A primer for morpholino
use in zebrafish.Zebrafish2009;6:69-77.
107. Justice MJ, Noveroske JK, Weber JS, Zheng B, Bradley A. Mouse ENU mutagenesis.
Hum Mol Gen1999;8:1955-1963.108. Chen Y, Yee D, Dains K, Chatterjee A, Cavalcoli J, Schneider Eet al. Genotype-
based screen for ENU-induced mutations in mouse embryonic stem cells.Nat
Gen2000;24:314-317.
109. Peterson RT, Link BA, Dowling JE, Schreiber SL. Small molecule developmental
screens reveal the logic and timing of vertebrate development.Proc Natl Acad Sci
USA2000;97:12965-12969.
110. Sachidanandan C, Yeh JR, Peterson QP, Peterson RT. Identification of a novel reti-
noid by small molecule screening with zebrafish embryos.PLoS ONE2008;3:e1947.
111. Cerutti S, Hoyer D, Voss A. Multiscale, multiorgan and multivariate complexity ana-
lyses of cardiovascular regulation.Philos Transact A Math Phys Eng Sci2009;367:
1337-1358.
112. Gehlenborg N, O"Donoghue SI, Baliga NS, Goesmann A, Hibbs MA, Kitano Het al.
Visualization of omics data for systems biology.Nat Methods2010;7:S56-S68.
113. Nakatsui M, Ueda T, Maki Y, Ono I, Okamoto M. Method for inferring and extracting
reliable genetic interactions from time-series